The U.S. electric infrastructure has essentially remained unchanged in its architecture for the past century. From an engineering perspective, this architecture has scaled remarkably well across the continent and has demonstrated impressive reliability, all things considered.
The classical centralized-generation grid architecture was designed toward the economies of scale and has achieved remarkably high effectiveness for that architectural paradigm. However, this architecture imposes efficiency and environmental ceilings because of the low penetration of renewable energy, lack of intelligent distribution, minimal (if any) storage, ad-hoc dispatch, uncontrolled load, and high distribution losses as power is moved large distances from generator to consumer. [1]
With a society increasingly reliant on electrical power, the once-good enough standard architecture based on centralized generation, arterial transmission and radial distribution is beginning to fall short for many critical applications. Microgrids offer a mechanism to intelligently compartmentalize the grid and provide additional focused measures for reliability where needed for absolutely critical loads, such as hospitals, data centers and military bases.
While definitions vary slightly from source to source, the United States Department of Energy’s definition of a microgrid puts it comprehensively and succinctly in the context of the broader electrical grid infrastructure. “A microgrid is a group of interconnected loads and distributed energy resources within clearly defined electrical boundaries that acts as a single controllable entity with respect to the grid; a microgrid can connect and disconnect from the grid to enable it to operate in either grid connected or islanded mode.” [2]
The U.S. Army’s RDECOM adds further specificity by stating that the microgrid is “capable to store, distribute, manage, import and export power, and interface with other relevant grids.” In its typical implementation, the microgrid is independently managed and maintains only a single Point of Common Connection to the broader utility grid. This concept, espoused strongly by the Consortium for Electric Reliability Technology Solutions (CERTS) early on, ensures that the microgrid can be seen by the broader grid not as a collection of multiple diverse distributed generators and loads, but as a single entity; one that can appear as a load, an energy resource or nothing at all when operating in island mode. [3] In grid-connected mode, the microgrid can consume or provide electricity to the utility.
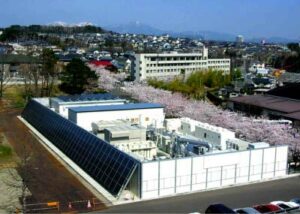
Example of a customer microgrid at Tohoky Fukushi University, Japan. (Courtesy of lbl.gov/Released)
The concerns associated with the ageing electrical infrastructure are not new to policy makers and system designers. The well-publicized Smart Grid initiatives strive to modernize the current “dumb grid” through high sensor density, improved communication networks, automated self-healing mechanisms and enhanced cyber security. In a fantastic symbiotic fashion, the Smart Grid and microgrids are able to provide significant mutual benefit. [4]
To the first degree, this is in the form of technology overlap, especially with respect to sensors, communications, power electronics and automated management systems. The second degree lies with the Smart Grid’s Advanced Metering Infrastructure (AMI), which includes a two-way communication path between the utility and consumer for usage, pricing and sensing. This has the potential to dramatically open up the electricity market such that microgrids can become direct economic players and dispatchable resources to the utility to aid automated healing.
Because of the vast scale of the existing electricity grid (over a terawatt across hundreds of thousands of miles of power lines), [5] retrofitting to a full smart grid that is self-healing, secure and full of renewables is a daunting task for utilities, both in terms of technology and cost.
However, by using a microgrid to integrate renewable generation resources in locally-managed fashion together with storage and inertial generation means that intermittency problems can be blunted before reaching the point of common connection to the grid. Because microgrids enable generation to be safely distributed across the grid, infrastructure upgrades along congested power arteries can be delayed. Thus, microgrids, which appear to the utility as self-contained loads/generators, much like the grid already has, are being perceived as handy ways to locally and modularly address needs to upgrade the grid, especially in the areas that need it most. [6]
Energy surety
The United States’ electric grid is among the most reliable in the world, yet there is a very good chance that you, the reader, have experienced a grid failure in the last 15 years. Move to a different country and that same cumulative likelihood of failure could exist over the past 15 days.
Guaranteeing the availability of electricity to critical loads is important enough that the buildings that house them are required to install their own backup generators and diesel tanks for times when the power goes out. These critical loads could include hospitals, emergency response centers, data centers, water pumping/filtration and operations centers for the military. The 2010 Quadrennial Defense Report defines energy security as “having assured access to reliable supplies of energy and the ability to protect and deliver sufficient energy to meet operational needs,” and this is a requirement for the electricity supply (United States DoD, Quadrennial Defense Review Report (2010)).
The grid is based on century-old technology and remains subject to the vulnerabilities inherent in its centralized architecture. Instabilities could result from any variety of natural disaster, accident, strike or act of terrorism. The most troubling examples are the outages that cascade from grid segment to grid segment as generators become overloaded and disconnect to maintain safety. One might recall the massive blackout across the U.S. Northeast in August 2003, which removed light for over 50 million people within eight minutes as 261 power plants tripped off. It cost the economy an estimated $10 billion by conservative measures. [7]
One could also recall the more recent outage on the opposite corner of the continental U.S. in 2011, which was caused by a single
human’s operator error but cascaded across the southwest. Even in local-outage cases, the results of a power failure can be dangerous.
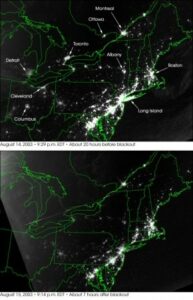
Before (top) and after (bottom) photos of a power failure that left many American cities in the dark on the evening of Thursday, Aug. 14, 2003. (Courtesy of NASA/Released)
For example, consider the heat wave of July 2006 when power to the FAA’s Los Angeles Air Route Traffic Control Center covering parts of Arizona, Nevada, Utah and California shut down. It could not restore radar and communications for an hour and a half while it waited for a backup generator to activate, effectively incapacitating Los Angeles International Airport. [8]
Islandable microgrids offer a mechanism for sites to add self-sufficient managed capacity for backup generation to prioritized loads in the case of grid failure. At the same time, microgrids provide a higher level of stability back to the grid as a whole by compartmentalizing distributed generation and managed loads.
In this scenario, a single generator failure is far less likely to cause a cascading failure across the entire grid; the microgrids can detect the instability and increase their output (or disconnect entirely), reducing the load on the remaining grid generators.
Microgrid technology is at least as important to the DoD as it is to any other customer. Some domestic bases experience power outages as often as 300 times per year, and forward-operating bases may need to operate without the luxury of any local grid support at all. [9]
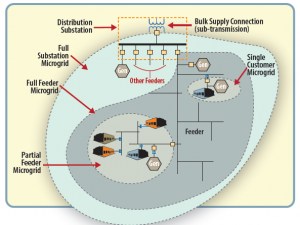
Diagram of the Energy Surety MicrogridTM developed by Sandia National Laboratory, which uses a new concept for energy generation and delivery systems. (Courtesy of Sandia National Laboratory/Released)
Backup diesel generators are the typical solution to the energy islanding need and work well for small installations (i.e., on a per-
building basis). Microgrids are designed and managed to efficiently enable islanding across multiple generation resources and multiple buildings that can span an entire large base.
The capability to integrate multiple types of generation on the same microgrid also addresses another concern related to energy surety, the availability of fuel. Multiple generators can run on multiple types of fuel (i.e., a natural gas turbine and diesel generator on the same microgrid), allowing diversification of the supply chain in times of supply volatility. Microgrids are typically designed with a mind toward integrating renewable resources, such as wind and solar, which may not require imported fuels at all. [8]
Net Cost Savings
Depending on the installation needs and the local utility environment, microgrids can in many cases save money over the course of a few years. There are three primary ways by which a microgrid can decrease operating costs, outlined as follows.
Generating power locally at a cost lower than the grid can supply it
Rooftop solar is now a familiar investment by businesses and consumers to offset their energy use and even sell low-marginal-cost power back to the grid via power purchase agreements with the local utility. Because microgrids can integrate those solar panels with generators, energy storage and other renewable resources in an intelligent, dispatchable fashion, they can increase the availability and quality of sellable power.
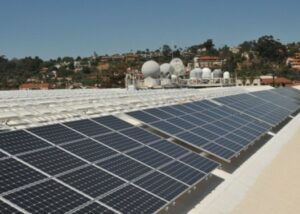
Solar panels installed on the roof of Space and Naval Warfare Systems Command Headquarters Old Town Complex in San Diego, California. (Photo by Rick Naystatt/Released)
The Advanced Metering Infrastructure (AMI) is already deployed across much of the United States and is equipped to perform near-real-time energy flow reporting to the utility and consumer via wired and wireless communications. Pending governing policy changes, this will likely more widely translate to real-time pricing structures per kilowatt-hour based on the current, live retail market price of energy. In this scenario, the microgrid has great flexibility in its choice of how to interact with the market. Its cost-based management algorithms can optimally balance its cost factors (efficiencies, capital depreciation, operations and maintenance, fuel, renewable resource forecast, storage cycling, etc.) and decide in near-real time whether to purchase or sell to the grid. It could feasibly even exercise energy arbitrage during periods of extremely high grid cost volatility.
Such energy demand/generation response also implicitly addresses the utility need for energy peak shaving, which serves to reduce the top capacity the grid infrastructure must be designed to handle. Large customers like military bases and factories are often charged additional infrastructure fees to offset the utility’s cost to stand up the fat distribution lines and substations needed to handle the large load peaks. By offsetting load peaks to the grid with local generation, the utility no longer needs the infrastructure-bolstering investment.
Selling ancillary services to the grid
Some energy services are even more valuable to the grid than active energy (i.e., kilowatt-hours). These are called ancillary services. The Federal Energy Regulatory Commission defines the ancillary services as “those services that are necessary to support the transmission of capacity and energy from resources to loads while maintaining reliable operation of the Transmission Service Provider’s transmission system in accordance with good utility practice.” [10] In order of value, the ancillary services worth most to the grid operator are regulation (voltage and frequency), spinning reserves and supplemental (non-spinning) reserves. [11]
The utility participates in high-value, fast-moving markets for these services, each of which can feasibly be provided by a microgrid. Regulation could be especially well-suited to be provided by inverters that can vary phase and frequency instantaneously. The ancillary services market attractiveness varies significantly from region to region, but it could prove a valuable income stream to the microgrid operator, especially as regulations move to support participation in these markets. [12]
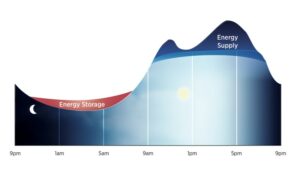
A graph of peak shaving showing the utility’s time-of-use rates. (Courtesy of http://en.openei.org/wiki//Released)
Improving efficiency of operations, especially when islanded
Microgrids allow significant optimization efficiency, both in grid-tied and islanded states. Consider even the specific simple case of traditional generators. Current backup generator systems are typically wired directly to critical load circuits, with a unique generator system for each building. This is not efficient use of fuel or capital, since each generator must operate at a very low load factor for the majority of operation time. If these multiple generators are networked across a broader microgrid instead, the load profiles are stabilized over a larger population. Now, generators can be selected and operated at optimal efficiency levels for their capacity with less volatility in load-tracking. Furthermore, redundancy in generators can be considered a shared resource across the entire microgrid instead of being required for each building; this reduces capital costs while maintaining the same reliability factor.
Forward-operating bases can be considered candidates of a special islanded-only application of microgrids. In recent conflicts, fuel resupply missions to these bases over mountainous terrain have been the cause of high casualty rates and high fully-burdened cost of fuel. When considering that electricity generation typically accounts for a large component of fuel use at camps and bases near the tactical edge, improving generation efficiency and integrating renewables via a microgrid architecture are highly valuable. [13]
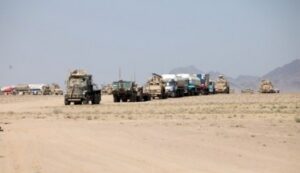
Marines from 2nd Maintenance Battalion, 2nd Marine Logistics Group (Forward), escort more than 35 local national trucks to forward operating bases in northern Helmand prov-ince, Afghanistan, during a resupply mission June 30 through July 6, 2011. (Photo by Sgt. Rachael Moore/Released)
Combined Heat and Power (CHP) technology may be a particularly attractive option for microgrid generation at fixed installations from
an efficiency standpoint. The efficiency of combustion generators is physically limited by the Carnot factor, which implies that waste heat will always be created. This waste heat can be captured and circulated to nearby buildings to serve many purposes, such as heating (air or water), cooling (via absorption processes) and air conditioning (via desiccation processes). By harvesting and distributing this heat energy directly, the total system efficiency (fuel conversion to electricity and heat to building) climbs from 45 percent to 80 percent.
Heat vs electricity tuning has been shown to achieve a further 2-4 percent efficiency gain. Because heat transmission is limited in how far it can go, CHP plants are most effective when located close to the heat receiver. Microgrids encourage distributed generation and make CHP a more valuable option where its benefits might be realizable in a traditional grid with distant transmission. CHP is forecast to grow to 1.9 GW of installed base to represent $7 billion by 2018. [14]
In any discussion about the cost savings achievable by microgrids, one must also consider the costs for deployment. Upgrading an installation to a microgrid infrastructure will require a significant amount of initial capital to cover components and labor. Anyone planning to install a microgrid should consider the financing options that have emerged to address this issue. Utilities will often negotiate Power Purchase Agreements or Energy Service Agreements up-front to ensure adequate payback forecasts across long-term contracts. Other multi-party financial instruments include Enhanced Use Leases, Renewable Energy Service Agreements or Utility Energy Service Contracts. Additional procedural hurdles and costs must be fully understood if crossing military/federal/state regulators. [15]
Environmental
Microgrids enable high-penetration of distributed renewable resources. Renewables, such as solar and wind, are intermittent in nature, and because of their low power density and siting requirements, they must often be geographically distributed. This presents a challenge to the traditional radial grid architecture, but microgrids have active control mechanisms and typically incorporate storage to offset intermittency effects. By connecting the microgrid’s locally-aggregated and stabilized renewable generation to the main grid via a controlled single Point of Common Connection, the grid is able to accommodate a higher penetration of renewables without the stability concerns typically caused by distributed renewables.
The National Defense Authorization Act of 2007 requires that 25 percent of all energy consumed from DoD installations be renewable by 2025, and many branches of the DoD have followed-up with their own energy efficiency and renewability goals. [16] Microgrids are an effective way to help meet or exceed these goals. This is important given the DoD’s vast electricity expenditures, $4
billion to power 300,000 buildings at 500 installations globally, and domestic bases will likely only increase demand as troops return home from conflicts abroad. [17]
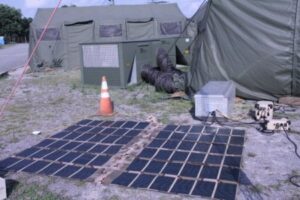
Microgrid systems are currently the only solution that allows the incorporation of multiple technologies, such as renewables and energy storage systems, to supplement traditional power generation techniques. (Photo by Spc. Robert Porter/Released)
Case Studies
For a detailed survey of 44 studied, planned or installed microgrids at DoD installations up through mid-2012, the reader is encouraged to read ref. [11]. This section will provide some updates to those reports and describe a few notable newer and non-DoD examples.
The Smart Power Infrastructure Demonstration for Energy Reliability and Security (SPIDERS) microgrid project led bySandia National Laboratories has completed phases 1-2 at Fort Carson, including a 72 hour plug-in electric vehicle demonstration. The next phase is at Camp Smith and will feature an entire cyber-secure 5 MW installation with high-penetration renewables, demand-side management and redundant backup. A co-developed product of the SPIDERS project is Sandia’s Microgrid Cyber Security Reference Architecture, which lists best practices for securing control systems in a microgrid. [15]
Forward Operating Bases may be important applications of microgrid technology in coming years. A recent simulation based on observed load data from a base in Afghanistan showed that microgrids powered by Advanced Medium Mobile Power Sources and multiple Tactical Quiet Generators can save 16 percent of fuel and reduce net operating hours by 54 percent, which reduces maintenance needs. [18]
The University of California, San Diego operates a large 42 MW microgrid with photovoltaic, fuel cell, and combined heat and power generation. It is considered one of the most efficient microgrids in the country, and the university reports saving $800,000 per month on energy expenditures after an initial investment of $8 million. The microgrid performed islanded operation during a large blackout event across Southern California, Arizona and Mexico in 2011. Princeton University has also demonstrated islanding of their smaller but similar 15 MW microgrid, which continued operating through outages caused by Superstorm Sandy in 2012, as did the Food and Drug Administration’s White Oak research facility, which has a large combined heat-and power plant. [19]
The Galvin Electricity Initiative has been active in microgrid demonstration projects, including demonstrators at Bella Coola, British Columbia and the Illinois Institute of Technology. The former demonstrated a 64 percent diesel use reduction by adding hydrogen storage to a diesel and hydroelectric microgrid. The latter includes real-time energy pricing communications to loads.
The Consortium for Electric Reliability Technology Solutions (CERTS) developed one of the first highly-publicized microgrids, which has become a model for the industry. The test bed incorporates synchronous and photovoltaic generation, load shedding, storage and intelligent energy management control. The microgrid has demonstrated it can achieve stable islanding and resynchronization without any high-speed communications between generators, and it is capable of handling inductive loads and internal faults. [20]
The Twenty-nine Palms Marine Corps Air Ground Combat Center microgrid installation introduced in the MIT Lincoln Labs study has since added 5 MW of photovoltaics, 500 kW of batteries, and a new 8 MW combined heat and power plant that accompany the existing plant. It continues to be successful in its mission of improved efficiency, reliability and environmental friendliness. [21]
Conclusions
Microgrids provide an architecture for electricity surety by enabling seamless transitions from grid-tied to self-sufficient islanded operation that can generate electricity from a variety of resources and fuels. Because they include an automated management element that communicates with its distributed resources and the utility in a location-based manner, microgrids can achieve a higher efficiency than is often possible with building-specific backup generators or even the conventional grid in many cases. Finally, in part because of that management element, microgrids enable the high-penetration insertion of renewable resources in a way that is not feasible with traditional backup systems or centralized grids.
Buoyed by investments in Smart Grid initiatives, microgrid technology has advanced rapidly in the past decade in technology readiness as simulations and lab demonstrations move to live pilot installations that support live critical loads. Some of these installations have had the opportunity to prove their worth when tested against real-world blackouts from weather-caused and utility-caused blackouts.
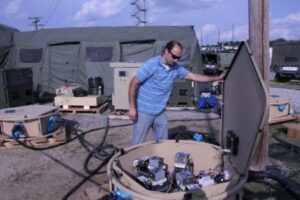
CERDEC demonstrated a proof of concept for a smart grid that could support tactical operations this summer at its integrated capabilities test-bed at Fort Dix, N.J. (Photo by Spc. Robert Porter/Released)
About the Author:
Mr. Chris Doran supports the HDIAC on behalf of Northrop Grumman Corporation to contribute subject matter expertise for Alternative Energy. He earned a B.S. in Electrical Engineering and an M.S. in Nanoelectronics with a focus on Energy Materials from the University of California, San Diego. His background includes laboratory research in nanoelectronics and flexible photovoltaics, as well as systems engineering for electric utility and defense industries.
References:
[1] Environmental Security Technology Certification Program. “Smart Microgrid Energy Management Controls for Improved Energy Efficiency and Renewables Integration at DoD Installations,” 2013.
[2] Department of Energy Microgrid Exchange Group.
[3] Consortium for Electric Reliability Technology Solutions (CERTS). “Integration of Distributed Energy Resources. The CERTS MicroGrid Concept. Consultant Report.” California Energy Commission, 2003.
[4] J. D. Guggenberger. “Performance Characterization And Optimization Of Microgrid-based Energy Generation And Storage Technologies.” Missouri University of Science and Technology, 2012.
[5] U.S. Department of Energy, Energy Information Administration, 2013.
[6] L. Tao, et al. “From Laboratory Microgrid to Real Markets – Challenges and Opportunities.” 8th International Conference on Power Electronics – ECCE Asia, 2011.
[7] R. W. Galvin, K. E. Yeager. “Perfect Power: How the Microgrid Revolution will Unleash Cleaner, Greener, and More Abundant Energy.” McGraw-Hill, Inc., 2008.
[8] S. B. Van Brockhoven, et al. “Technical Report 1164. Microgrid Study: Energy Security for DoD Installations.” Lincoln Laboratory Massachusetts Institute of Technology, 2012.
[9] M. Hightower. “Energy Surety Microgrids™ for Critical Mission Assurance to Support DOE and DoD Energy Initiatives.” Energy Systems Analysis Department, Sandia National Laboratories.
[10] FERC Order 888-A, April 1996.
[11] Y. Xiao (ed). “Communication and Networking in Smart Grids.” CRC Press. 2012.
[12] Federal Energy Regulatory Commission Staff. “Payment for Reactive Power Commission Staff Report,” 2014.
[13] Army Environmental Policy Institute. “Sustain the Mission Project: Casualty Factors for Fuel and Water Resupply Convoys Final Technical Report,” 2009; P. Asmus, K. Adamson. “Military Microgrids Stationary Base, Forward Operating Base, and Mobile Smart Grid Networks for Renewables Integration, Demand Response, and Mission-Critical Security.” Pike Research, 2012.
[14] Reliability Information Analysis Center (RIAC). “Assessment of Microgrid Applications to Tactical Edge Military Operations.” Technical Report: RIAC TAT Number – RI-12-RMS#117/DO#203, 2013.
[15] C. K. Veitch, et al. SAND2013-5472 “Microgrid Cyber Security Reference Architecture.” Sandia National Laboratories, 2013.
[16] Environmental and Energy Study Institute. “DoD’s Energy Efficiency and Renewable Energy Initiatives,” 2011.
[17] Installations and Environment. “Department of Defense Annual Energy Management Report Fiscal Year 2013,” 2014.
[18] U.S. Army Materiel Systems Analysis Activity. Technical Report No. TR-2014-04. “AMSAA Analysis of Project Manager Mobile Electric Power Operational Energy Solutions in Afghanistan “Operation Dynamo II,” 2014.
[19] J. St. John. “How Microgrids Helped Weather Hurricane Sandy.” Greentech Media. https://www.greentechmedia.com/articles/read/how-microgrids-helped-weather-hurricane-sandy, 2012.
[20] J. Eto, et al. “Overview of the CERTS Microgrid Laboratory Test Bed.” CIGRE2009 (2009); CERTS Microgrid Phase Two Test Report.
[21] U.S. Department of Defense Environmental Security Technology Certification Program. ESTCP Cost and Performance Report (EW-200937): “Smart Microgrid Energy Management Controls for Improved Energy Efficiency and Renewables Integration at DoD Installations,” 2013; K Kaufmann. “Twenty-nine Palms co-gen plant aims for efficiency.” The Desert Sun. 30 March 2014. http://www.desertsun.com/story/tech/science/energy/2014/03/29/twentynine-palms-co-generation-power-plant/7069857/.