As warfare technology has progressed to more sophisticated and deadly tactics, the prevalence and severity of traumatic injury have increased. During Operation Iraqi Freedom and Operation Enduring Freedom, 78 percent of wounds sustained in combat were a consequence of explosions, and extremities were the most commonly affected body region. [1] Due to the severity and frequency of these types of injuries, surgeons working as part of forward surgical teams must think, move and act more quickly to keep pace with patients’ needs because mistakes could result in major tissue loss or death.
A study investigating both the frequency and nature of injuries treated by an FST in Afghanistan during the initial phase of Operation Enduring Freedom found that debridement was the most commonly performed procedure. [2] Debridement is the removal of entire sections of damaged tissue, while retaining as much healthy tissue as possible. This process relies on surgical expertise and includes visual and tactile inspection.
Unfortunately for surgeons operating as part of FSTs, extensive wound assessment and treatment options can be restricted, while the clock is ticking on saving tissue and lives. Finding a balance between removing and keeping tissues can mean the difference between risking life-threatening infection and saving a limb. Proper wound debridement is critical for the subsequent wound healing processes, but the current methods for assessment lack precision and require too much time. Injuries to extremities can be expected to persist as the use of explosives in combat continues to rise. Wound assessment will remain a time-consuming and complicated treatment step if new methods are not implemented.
To accommodate the need for more precise treatment, new surgical techniques can be developed to incorporate fluorescent probes that assist surgeon assessments of wounds. By using fluorescently-labeled tags to target tissue that needs to be removed, a surgeon can create a clear and distinct visual boundary between nonviable and healthy tissue. These advances would not
only alleviate time pressure but would also improve the quality of care given to patients by establishing a quantifiable method for zone of injury determination.
One prospective option is the use of a biocompatible chemical probe, which may be achieved based on the unique properties of the peptide pHLowInsertionPeptide. The name pHLIP appropriately describes the peptide’s mechanism: an increase in the rate of glycolysis within the cells of injured tissues causes a lowered local pH environment, which allows the peptide to insert into cell membranes. pHLIP exists in three conformational states. The first is the free-floating monomeric peptide in solution. If tissue at a physiological pH (~7.4) is exposed to pHLIP, the peptide adopts a conformation that allows it to loosely associate with the cellular membranes that are made up of lipids. When the pH dips below physiological levels, pHLIP assumes a coiled conformation and inserts across the lipid membrane. This characteristic presents a unique opportunity to use pHLIP to monitor any cellular process involving a decrease in the local pH
environment, including cancer, tissue damage and more. [3,4,5,6]
Localized acidity targeting is achieved through a relatively simple process. A pH drop causes protonation of aspartic acid and glutamic acid residues of the peptide, increasing the peptide’s affinity for lipid bilayers and promoting insertion. [7] Upon insertion, the C-terminus penetrates the cell while the N-terminus remains outside. Researchers can capitalize on this process by attaching fluorescent tags to the N-terminus of the peptide, allowing real-time monitoring of cellular perturbations. In particular, pHLIP can be used to visualize the zone of injury and differentiate between viable and damaged tissue based upon simple pH changes.
While using pHLIP conjugates to identify wound boundaries is novel, pHLIP-fluorophore conjugates have been extensively studied for early detection of cancer and to improve surgical tumor removal. [3,4] The pHLIP-conjugated fluorescent response is directly related to extent of injury, as more serious tumors cause more acidic local environments due to the localized increase in the rates of glycolysis of those tissues. This increased glycolysis will decrease the local pH and ultimately increase the peptide concentration on the tumor surface.
Further, fluorescence can be measured and quantified to improve diagnosis, as targeting changes in glycolysis has been directly correlated with the aggressiveness of tumors. [3] To determine the relationship between fluorescent signal and malignancy, Reshetnyak et al. found higher concentrations of fluorescent pHLIP in tumors with necrotic and apoptotic cores than in tumors with no necrotic tissue. [6] As necrosis is a hallmark of wounds, the same correlation is expected in cases of wound healing. The scope of pHLIP cancer research creates a well-established theoretical base from which researchers can build to expand pHLIP use to wounds.
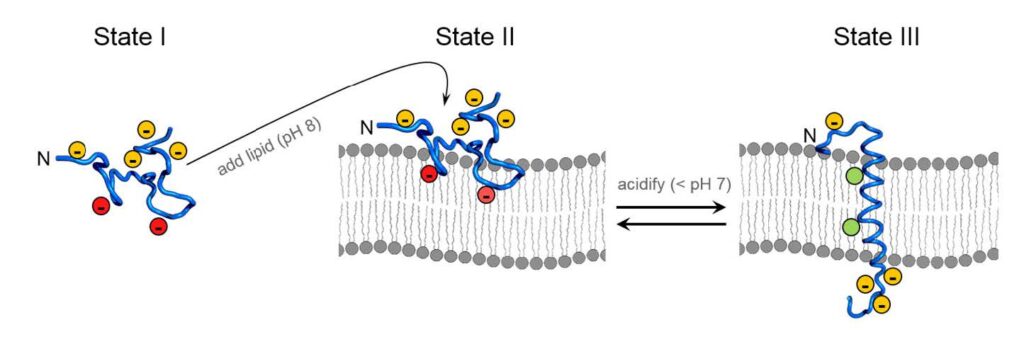
Figure 1. Biophysical Mechanism of pHLIP insertion. State I is the free-floating, monomeric peptide with deprotonated Asp and Glu residues; red designates the Asp and Glu located in the conserved Leucine coil region. State II illustrates that at physiological pH, the peptide will associate with lipid membranes in solution but remains unstructured. State III is the structured peptide inserted as an α-helix across the lipid bilayer. (Modified with permission from Blake Mertz, Ph.D./Released)
Fluorescent tools like pHLIP can probe injuries below the tissue surface, and the only criteria for insertion is an acidic environment. Once it targets this region by inserting into diseased or damaged cells, the excess pHLIP conjugate can be distributed and excreted, and only an illuminated zone of injury will remain. After a short clearance time, clinicians can repeatedly use pHLIP
conjugates to monitor patient progress and predict the course of treatment.
Wound healing is a complex biological process, beginning with hemostasis and the formation of a platelet plug, then moving to inflammation, when a variety of immune signals are recruited to the wound to prevent infection. During the next phase, cell proliferation, new tissue grows to eventually cover the wound. Finally, the new tissue fully differentiates and matures, which increases
the resilience of the closed wound. Complete wound healing can take up to two years, so it is critical that surgeons monitor this delicate process to ensure chronic wounds or infection do not develop.
Wound assessment is an integral part of healing success, and clinicians must evaluate the tissue types, check for presence of inflammation and determine the wound edge. Of these criteria, determining wound edge is the most elusive because there are no well-developed methods to quantify the extent of a wound. Removing the dead or dying tissue is an essential step in initiating
wound healing, and successful excision of diseased tissue largely impacts the quality of care. In fact, a study by Cardinal, et al. confirmed a strong correlation between performing surgical debridement and improved wound healing rates, showing that in venous leg ulcers and diabetic foot ulcers, debridement procedures improved wound closure progression. [8]
Unfortunately, improper debridement can result in necrotic tissue remaining at the injured site, providing optimal growth conditions for bacteria and creating a nidus for infection. Complete debridement is important for treating abscesses that, when improperly treated, can develop into infections and cause sepsis. A purely visual estimation of wound edge takes more time and expertise, while increasing the risk of leaving diseased tissue behind. On the other hand, delayed wound healing can also be a consequence of over-debridement, where too much tissue is removed from the injured site. Finding a happy medium between these cases is challenging, and FSTs must make these complicated decisions regularly.
Currently, there are no standardized guidelines for surgical debridement. A certain amount of surgical expertise and time are involved, requiring years of experience and often consultation with other experts. Additionally, attempting to make such an evaluation under pressure can lead to inadequate debridement. This risk necessitates the development of a new tool to assist FSTs in
treating injuries sustained on the battlefield. Using pHLIP for more precise debridement would not only drastically improve patient success, but it would also prevent situations where incomplete debridement introduces new complications for patients.
Following a traumatic injury on the battlefield, FSTs have many decisions to make in a short time period. The high frequency of severe wounds requires FSTs to spend a large amount of their limited resources managing these difficult injuries. The development of fluorescent pHLIP diagnostics would provide FSTs a novel tool to help them make quick decisions in the field regarding
wound severity and treatment strategies.
To this end, researchers have simulated localized injuries in cell culture and exposed them to pHLIP-fluorophore conjugates that allow for perspicuous visualization. Specifically, L6 rat skeletal muscle myoblasts, which are optimal for emulating the conditions of a wound, grown and fully differentiated into fibroblasts that have the characteristics of smooth muscle tissue, were exposed to pHLIP-1-FITC conjugates under varying acidic or basic environments. The acidic conditions are essentially a simulated injury representative of a wound, while basic environments can serve as a
control to ensure that pHLIP will not insert into the membrane at higher pH values.
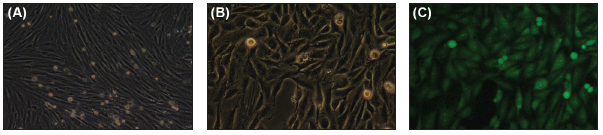
Figure 2. Induced injury in L6 cells results in strong fluorescent signals. Cells in (a) are normal, differentiated L6 cells before any exposures. L6 myoblasts were differentiated by decreasing serum levels in growth media from the typical 10 percent to 2 percent, as in Lawson . [9] Full differentiation took approximately 72 hours. Cells were exposed to 70 percent methanol to induce cell death. Fluorescently-labeled pHLIP was introduced at a concentration of 0.5μM and pH was monitored. When the pH dropped below pH = 6.5, pHLIP insertion became apparent as shown in (c), where one can see distinct green fluorescence. As expected, this fluorescence created a visualization of injury that could be seen easily under the fluorescent microscope. These pictures show the same frame in (b) normal light and (c) under a fluorescent lens. (Released)
important to study how the slight sequence variation could affect aggregation, as the primary goal of this research is to develop an injectable product.
Once a well-developed system has been established, it will be possible to build on this knowledge to create a variety of products for different clinical applications. pHLIP technology allows for this type of flexibility by changing the tag on the peptide. While not currently feasible, this technique would have the greatest impact if it could be detected with the naked eye, rather than a fluorophore, and be used practically anywhere. Imagine if FSTs were able to evaluate the severity of wounds in real time on the field, with nothing other than a simple injection upstream of the injured area.
In the meantime, FSTs can employ UV fluorescent or NIR fluorescent dyes, and there are advantages to each. A fluorescent dye like fluorescein provides a cheap, easy-touse product that could be transported and used almost anywhere. NIR fluorescent dyes, like Indocyanine Green can be used for more sensitive detection, as they reduce the amount of white light interference and background interference from biological sources. Its use would require implementing already existing technologies in hospitals to image the NIR signal. With either product, the goal is an injectable solution of pHLIP that can be rapidly visualized.
pHLIP products are not limited to wound debridement alone and can potentially develop into applications for in situ wound treatment. Drug delivery capabilities of pHLIP have been investigated by several groups, demonstrating the peptide is capable of delivering cell-impermeable cargo across the plasma membrane. pHLIP has delivery potential through another mechanism, as it can also carry cargo by tethering small molecules to the plasma membrane surface. This is the principle behind using the attached fluorescent probes for injury site identification.
Drug delivery feasibility is often guided by Lipinski’s rule of five, which suggests drugs should be small and hydrophobic to reach intracellular targets. Many biological inhibitors cannot pass through a membrane unassisted, but pHLIP can translocate these agents with ease. pHLIP has successfully been shown to deliver gold nanoparticles to tumor sites via intravenous injection, allowing for “controlled” toxicity to tumors. [12]
More traditionally, it can inject and release small molecules inside the cell. When small molecules are attached to the C-terminus of the peptide, they are transported inside the cell. The reducing environment of the cytosol results in bond breaking, triggering a release of the cargo. A study of the translocation of phalloidin, a toxic agent that inhibits tumor growth, confirmed the ability of the peptide to effectively deliver cargo. When the toxin was attached to the C-terminus and exposed to cells in an acidic environment, it prohibited tumor proliferation. [13] These findings confirmed pHLIP viability for drug delivery.
Considering the drug delivery capabilities of pHLIP, one can begin to envision the possible applications in wound healing and treatment. pHLIP has already been shown to transport cell-impermeable molecules across plasma membranes to treat tumors [13], so it is not a far reach to apply this technology to wounds. For drug delivery applications, cell-impermeable cargo can be attached to the peptide’s C-terminus, as previously stated. The cargo is attached via a disulfide bond that can subsequently be cleaved upon insertion, releasing the cargo into the cytoplasm where it can act therapeutically. [6]
One of the most challenging combat injuries that FSTs face is deep bleeding wounds, such as large chest cavities. These types of wounds cannot be treated with a tourniquet and result in major blood loss. Currently, there is no way to deal with this deep bleeding other than to physically restrict blood flow. Cauterizing and pinching off vessels are the only options but are usually ineffective,
as it is extremely difficult to deal with the extent of the cavity. [14] pHLIP peptides could be used to introduce coagulating agents to essentially pinch off arteries and create a sort of molecular tourniquet.
For example, during the inflammatory phase of wound healing, peroxisome proliferator- activated receptor β acts as an anti-apoptotic factor, so survival of cells in the affected area depends on its activation. [15] Peptide delivery of activating factors early on can potentially provide a way to induce wound healing processes at the cellular level earlier than the body would normally be able to accomplish. This application of pHLIP can only be accomplished if researchers understand the steric factors involved, as well as the dynamics of pHLIP that allow it to localize to injured tissues and insert in a few seconds.
Therefore, this type of treatment could act quickly to prevent major blood loss and assist the body’s efforts to begin wound healing. Since all pHLIP peptides insert at low pH, it would be possible to deliver a cocktail of different agents to the site, including anti-bacterial and anti-inflammatory agents to reduce the severity of the injury, further assisting the wound healing process. However,
there is much work to be done before these types of therapies can be developed.
FSTs face a different set of challenges than a typical operating room, encountering patients whose injuries are so severe that they cannot be transported to a care facility without immediate surgical intervention. One of the challenges faced by FSTs is the pressure to make quick decisions about wound treatment in a short amount of time, but current methods involve time-consuming treatment by highly trained and experienced clinicians. Fluorescent pHLIP products could free up resources by cutting down on time spent for debridement evaluation.
A shift to this technology will allow more confident decisions to be made by FSTs regarding debridement, leading to an increase in tissue preservation. Furthermore, the technique is very intuitive, as it will be administered by simple intravenous injection. FST surgeons would not need excessive training to become familiar with its use, and it will be integrated easily into existing technologies. Therefore, it will not impose high costs for transition.
pHLIP technologies provide promising possibilities for applications in the field by creating new ways to quickly assess injuries, and the key to effective treatment is adaptability to multiple situations. Additional research aimed at deep tissue injuries may lead to an affordable, easy-to-transport tool that is flexible enough to assist in a widerange of medical cases. Different peptide
variants can be designed with a variety of fluorophores or cargo molecules to meet the specific needs for many of the disparate circumstances faced by FSTs and general military medicine.
By developing pHLIP conjugated products, physicians will gain access to new treatments for combat wounds, spanning from more precisely identifying the wound edge for debridement to delivery of wound-healing factors that initiate the healing process. This improved accuracy and broadened scope will lead to tissue preservation, decreased rates of infection, enhanced quality
of care and better patient outcomes.
References
1. Owens, B. D., Kragh, Jr., J. F., Wenke, J. C., Macaitis, J., Wade, C. E., & Holcomb, J. B. (2008). Combat wounds in Operation Iraqi Freedom and Operation Enduring Freedom. The Journal of Trauma Injury, Infection and Critical Care, 64(2), 295-299. Retrieved from https://www.ncbi.nlm.nih. gov/pubmed/18301189 (accessed January 17, 2017)
2. Peoples, G. E., Gerlinger, T., Craig, R., & Burlingame, B. (2005). Combat casualties in Afghanistan cared for by a single forward surgical team during the initial phases of Operation Enduring Freedom. Military Medicine, 170(6), 462-468. Retrieved from http://dx.doi.org/10.7205/MILMED.170.6.462 (accessed January 17, 2017)
3. Segala, J., Engelman, D.M., Reshetnyak, Y.K., & Andreev, O.A. (2009). Accurate analysis of tumor margins using a fluorescent pH Low Insertion Peptide (pHLIP). International Journal of Molecular Sciences, 10(8), 3478-3487. Retrieved from http://dx-.doi.org/10.3390/ijms10083478 (accessed January 17, 2017)
4. Weerakkody, D., Moshnikova, A., Thakur, M. S., Moshnikova, V., Daniels, J., Engelman, D. M., … & Reshetnyak, Y.K. (2013). Family of pH (low) insertion peptides for tumor targeting. PNAS 110(15), 5834-5839. Retrieved from http://dx.doi.org/10.1073/pnas.1303708110 (accessed January 17, 2017)
5. Andreev, O. A., Engelman, D. M., & Reshetnyak, Y. K. (2009). Targeting acidic diseased tissue: New technology based on use of the pH (Low) Insertion Peptide (pHLIP). Chimica Oggi – Chemistry Today, 27(2), 34-37.
6. Reshetnyak, Y. K., Yao, L., Zheng, S., Kuznetsov, S., Engleman, D. M., and Andreev, O. A. (2011). Measuring tumor aggressiveness and targeting metastatic lesions with fluorescent pHLIP. Molecular Imaging and Biology, 13(6), 1146-1156. Retrieved from http://dx.doi.org/10.1007/s11307-010-0457-z (accessed January 17, 2017)
7. Andreev, O. A., Engelman, D. M., & Reshetnyak, Y. K. (2010). pH-sensitive membrane peptides (pHLIPs) as a novel class of delivery agents. Molecular Membrane Biology,
27(7), 341-352. Retrieved from http://dx.doi.org/10.3109/09687688.2010.509285 (accessed January 17, 2017)
8. Cardinal, M., Eisenbud, D. E., Armstrong, D. G., Zelen, C., Driver, V., Attinger, C., … & Harding, K. (2009, May 20). Serial surgical debridement: A retrospective study on clinical outcomes in chronic lower extremity wounds. Wound Repair and Regeneration, 17(3), 306-311. Retrieved from http://dx.doi.org/10.1111/j.1524-475X.2009.00485.x (accessed January 17, 2017)
9. Lawson, M. A. & Purslow, P. P. (2000). Differentiation of myoblasts in serum-free media: Effects of modified media are cell line-specific. Cells Tissues Organs 167(2- 3), 130-137. Retrieved from http://dx.doi. org/10.1159/000016776 (accessed January17, 2017)
10. Fendos, J., Barrera, F. N., & Engelman, D. M. (2013). Aspartate embedding depth affects pHLIP’s insertion pKa. Biochemistry52(27), 4595-4604. Retrieved from http://dx.doi.org/10.1021/bi400252k (accessed January 17, 2017)
11. Karabadzhak, A. G., Weerakkody, D., Wijesinghe, D., Thakur, M. S., Engelman, D. E., Andreev, O. A., … & Reshetnyak, Y. K. (2012). Modulation of the pHLIP transmembrane helix insertion pathway. Biophysical Journal, 102(8), 1846-1855. Retrieved fromhttp://dx.doi.org/10.1016/j.bpj.2012.03.021 (accessed January 17, 2017)
12. Yao, L., Daniels, J., Moshnikova, A., Kuznetsov, S., Ahmed, A., Engelman, D. M., … & Andreev, O. A. (2013). pHLIP peptide targets nanogold particles to tumors. PNAS, 110(2), 465-470. Retrieved from http://dx.doi.org/10.1073/pnas.1219665110 (accessed January 17, 2017)
13. Wijesinghe, D., Engelman, D. M., Andreev, O. A., & Reshetnyak, Y. K. (2011). Tuning a polar molecule for selective cytoplasmic delivery by a pH (Low) Insertion Peptide. Biochemistry, 50(47), 10215-10222. Retrieved from http://dx.doi.org/10.1021/bi2009773 (accessed January 17, 2017)
14. Hunt, P. A., Greaves, I., & Owens, W. A. (2006). Emergency thoracotomy in thoracic trauma- a review. Injury, 37(1), 1-19. Retrieved from http://dx.doi.org/10.1016/j.injury.2005.02.014 (accessed January 17, 2017)
15. Montagner, A., Wahli, W., & Tan, N. S. (2015, April). Nuclear receptor peroxisome proliferator activated receptor (PPAR) β/δ in skin wound healing and cancer. European Journal of Dermatology, 25(Suppl. 1), 4-11. Retrieved from http://link.springer.com/article/10.1684/ejd.2014.2505 (accessed January 17, 2017)