Space Situational Awareness (SSA) is the predictive knowledge and characterization of natural and/or synthetic resident space objects (RSOs) and the broader operational environment upon which space operations depend [1]. Space surveillance systems contribute to SSA by employing multiple sensor networks to detect, characterize, and track RSOs. Many of these resident space objects represent a significant threat to U.S. communication and surveillance systems, in part because an estimated majority of these objects remain undetected.
As space systems use continues to increase, navigation and operation are likely to grow more difficult as additional traffic and debris contribute to congestion. Government organizations, non-governmental organizations, and private firms alike are seeking to gain and advance space capabilities, making SSA increasingly critical as space steadily becomes more crowded and contested.
Overview
An RSO can be a fabricated object, such as a satellite, spacecraft, or debris, or a natural object, like an asteroid or meteoroid. There are currently more than 23,000 confirmed trackable objects present in low Earth orbit (LEO) and geosynchronous Earth orbit (GEO), according to the U.S. Space Surveillance Network (part of the Joint Space Operations Center, U.S. Strategic Command) [2]. These objects weigh an estimated total of 7,500 tons [3]. Most of these RSOs were cataloged by tracking them throughout operation, or from observed fragmentation events. According to the Joint Space Operations Center, of those 23,000 objects, roughly 4,800 are satellites, with slightly more than a quarter of those being operational [2].
With only approximately 1,700 operational satellites in orbit [4], the vast majority (by count) of RSOs orbiting our planet are types of debris. Based on statistical modelling and estimation derived from observations and analysis, there are more than 29,000 RSOs that measure greater than 10 centimeters (cm) in length; more than 750,000 objects measuring between 1 and 10 cm, and more than 166 million objects smaller than 1 cm [5]. This debris can originate from space missions that intentionally discard certain objects or parts during delivery and operations [6]. This type of RSO includes objects such as empty fuel/propellant tanks, separation and packaging equipment, payload shrouds, and even lens caps [6]. Other debris can be attributed to failure and fragmentation events that occur in orbit.
Fragmentation consists of in-orbit object breakups—more than 200 objects have experienced a fragmentation event since the first recognized orbital fragmentation event occurred in June 1961 [6]. Spacecraft also typically deteriorate over time, leading to gradual breakup of the craft, which is referred to as “anomalous debris [6].”
In addition to debris tracking and monitoring, SSA also involves the detection, characterization, and tracking of all orbital launches and the subsequent tracking of their payloads once in orbit. Synthetic RSOs can pose both intentional and unintentional threats, and being able to distinguish between the two is imperative for homeland defense [1]. Adversarial entities continually seek to develop capabilities aimed at hampering U.S. space operations [1], making the ability to monitor and track all payloads vital to national security by assuring the Department of Defense’s (DoD) continued operational security in space.
The sheer number of objects orbiting our planet is considerably large, and these objects have the potential to cause significant damage to spacecraft and space operations. According to the National Aeronautics and Space Administration (NASA), the International Space Station has been forced to conduct 25 avoidance maneuvers in its 20 years of operation [4]. Additionally, NASA reported 21 avoidance maneuvers by unmanned spacecraft in 2017 alone [4]. These avoidance maneuvers can only be initiated for known objects, which are typically larger objects. No reliable capabilities currently exist for detecting objects smaller than 10 cm, which, according to NASA, pose the highest penetration risk to the majority of operational spacecraft [4].
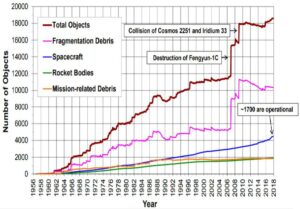
Figure 1. Rough approximation of all known objects in Earth orbit 10cm or larger [25].
Highlighting that threat, current collision avoidance detection and maneuver activities are at present conducted only for tracked objects, which constitute less than 1 percent of all potentially mission-ending orbital debris threats [4]. This leaves a number of critical commercial and government systems at a high level of risk for impact or attack. This includes nearly all commercial communications systems, such as radio and television assets, as well as several mission-critical military and government systems including the Military Global Positioning System Augmentation System [7] and Wide Area Augmentation System (WAAS) [8]. The WAAS is a satellite navigation system operated by the Federal Aviation Administration that provides service to all classes of aircraft, including vertically-guided landing approaches, meteorological conditions, route navigation, and airport departures and arrivals [8].
Perhaps the most critical system at risk is the network of missile defense satellites located in GEO. One such system is the Space-Based Infrared System operated by Air Force Space Command (AFSPC), which is designed to “meet jointly defined requirements of the defense and intelligence communities in support of the missile early warning, missile defense, battlespace awareness, and technical intelligence mission areas [9].” The Geosynchronous Space Situational Awareness Program satellites, tasked with supporting U.S. Strategic Command (USSTRATCOM) space surveillance operations, are also at risk from RSO impacts [10].
RSO Detection
Detection of any object in space can be a difficult task, given the scope and nature of the problem. Nevertheless, space- and groundbased optical and radar measurements have proven to be viable detection methods [11]. However, these methods are mostly limited to the detection of objects larger than 10 cm [11]. In GEO, specifically, these methods are exceptionally poor at detecting objects that are small in size or present only faint electromagnetic signals [11]. This is due to the inverse square law, which holds that signal source intensity is inversely proportional to the square of the distance. This means that by the time a radar signal has travelled to an object and reflected back to the detector, the signal intensity will be inversely proportional to the fourth power for the range to target. At GEO altitudes—approximately 22,000 miles up—RSO detection capabilities are largely nonexistent [11]. In fact, above LEO altitudes of roughly 1,240 miles, any object smaller than 10 cm is essentially undetectable [4]. For objects around 1 millimeter in size, there is currently no technology capable of detection at any altitude [4].
While USSTRATCOM, AFSPC, and NASA consistently operate and update space surveillance systems for RSO activity [2], the detection and identification capabilities of these systems need to keep pace with the increasing threat presented by the growing orbital landscape. According to researchers at the Guggenheim School of Aerospace Engineering at the Georgia Institute of Technology, approximately 8 percent of cataloged RSOs reside in GEO-like orbits [11]. Fundamentally, the quantities, types, sizes, and orbits of the vast majority of these objects are relatively unknown, excluding consistently bright and relatively stable-orbit objects [11]. This is in part due to their size, reflectivity, or high area-to-mass ratio that makes them particularly susceptible to orbital perturbation [11].
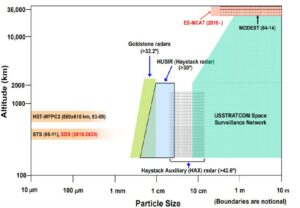
Figure 2. SSA Coverage for the United States. Shows coverage areas of space debris observation capabilities and large gaps in coverage area [25].
As reported by a research team from the Air Force Institute of Technology, many of the algorithms currently employed to detect RSOs use a “matched-filter or spatial correlator on long-exposure data to make a detection decision at a single pixel point of a spatial image based on the assumption that the data follow a Gaussian distribution [12].” Long-exposure imaging in daylight conditions requires stacking numerous short-exposure images in order to avoid overexposure, which provides an opportunity to increase detection capabilities [12]. Their idea involves developing an algorithm that drastically improves the traditional set of images by “selectively removing short-exposure frames of data that do not positively contribute to the overall signalto-noise ratio of the averaged image [12].” Cleaning the image stack in this fashion has the potential to extend RSO detection capabilities for smaller and dimmer objects.
Other promising avenues for increased RSO detection include using streak observations with advanced image processing techniques and computational abilities to increase optical detection of RSOs from a space-based platform [13]. Additional efforts involve using networked image sensors to determine angular velocity associated with an object and identifying a direction of motion to initiate a search for the possibly identified object [14].
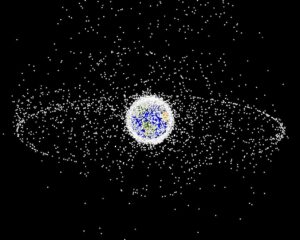
Figure 3. “The GEO images are images generated from a distant oblique vantage point to provide a good view of the object population in the geosynchronous region (around 35,785 km altitude) [26].”
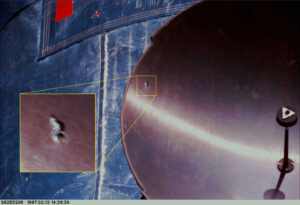
Figure 4. An impact that completely penetrated the antenna dish of the Hubble Space Telescope
[26].
RSO Characterization
In addition to detecting RSOs, it is equally important to be able to characterize detected objects. Characterization of RSOs is what separates our awareness of a satellite RSO from space debris RSO, and it is what determines which RSOs may pose a threat. Determination of shape, size, makeup, and orbital dynamics are also crucial in classifying satellite types. Within the context of space arms control, treaties currently in force prevent the placement of weapons of mass destruction in orbit, but do very little to restrict other types of platforms or satellites [15]. Some national security analysts estimate that space could be weaponized by early 2020 [15], meaning that technology and techniques to accurately characterize and classify newly detected objects will need to be established now to maintain an appropriate level of intelligence and security in the future.
One novel solution put forward by researchers at the Air Force Office of Scientific Research (AFOSR) suggests an integrated approach involving continuous variables to characterize space objects through joint search, detection, and classification, along with tracking problems previously deemed too computationally intense [16]. This approach to overcoming computational hurdles involves using artificial intelligence and machine learning techniques that follow autonomic approaches [16].
One such technique includes the use of methods based on dynamic data-driven application systems for the approximation of solutions to the Finite Set Statistics (FISST) recursion equations using randomized Markov chain Monte Carlo algorithms and Gaussian Mixture models [16]. The goal of this AFOSR project is to maximize characteristic information regarding space objects, which will in turn lead to a higher level of SSA.
Fellow researchers at AFOSR are taking a different approach by studying advancements in image analysis and applications involving 3D imaging and characterization [17]. The project’s objectives include the enhancement of the computational speed of Bayesian error estimations; the development of spectropolarimetric modeling of RSOs to extract dimensional and material characteristics; the enhancement of highly turbulent atmospheric modeling; and the production of phase-masks to allow 3D imaging of RSOs [17]. These would provide a full characterization of any detected RSO, allowing for object classification. Beyond classifying an object as a satellite (versus debris, etc.), it would also provide critical details to the intent or mission objective of any payload delivered by another nation. Using an object’s detailed 3D image along with its material characteristics, differentiating a surveillance satellite from a possible weapons platform or navigation satellite is likely to become decidedly easier.
RSO Tracking
Tracking RSOs can be difficult due to multiple sources of uncertainty, which include but are not limited to varied data sources, orbital mechanics, and object kinematics. The high cost and amount of resources required to achieve the direct, active monitoring of all RSOs with current technology makes it an impractical task. Because the bulk of RSO tracking is currently conducted by calculating orbital paths (not via direct monitoring), effective tracking of the majority of RSOs requires precise prediction of orbital characteristics and identification of alterations to orbital parameters. Improving prediction capabilities will allow for a higher level of safety and security for both spaceflights and satellite operations while navigating through orbit.
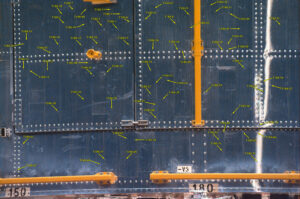
Figure 5. After in space repairs to the Hubble Space Telescope, the returned parts show many orbital debris impacts [26].
There are numerous other projects underway at AFOSR that aim to solve the RSO problem. For example, one effort is deploying FISST methodology on non-Euclidean manifolds with the goal of detecting, tracking, identifying, and characterizing multiple RSOs simultaneously [20]. Alternatively, another project is developing analytical and computational tools to improve tracking via the “optimal planning and scheduling of disparate sources of information, integrating sensing with stochastic models, uncertainty characterization and forecasting, and integration of sensor data with model predictions [21].”
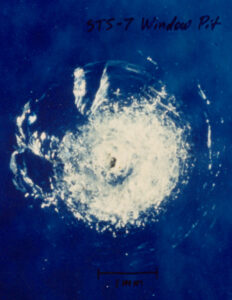
Figure 6. Window pit from orbital debris on
STS-007. Upon examination of the impact,
it was determined that the impacting object
was approximately 0.01cm in diameter and
travelling roughly 5km/s [26].
Other solutions involve a significant boost to data processing capabilities in addition to increased monitoring capabilities. With an increase in optical data there follows the need for faster optical data processing. The use of directional statistics could provide that increase. By using Fisher-Bingham-Key distribution for “observation-to-track association of angles-only optical data [24],” researchers at Applied Defense Solutions have shown a better data association than standard approaches that are more prone to Type II errors [24]. As described, this is “desirable in SSA scenarios when the modeled dynamics deviate from the true dynamics or in scenarios with unknown optical measurement biases [24].”
Conclusion
Significant research and technical development, including increased pattern recognition, real-time optical abilities, and computational algorithms for real-time classification, goes into the detection, tracking, and characterization of RSOs. These capabilities play a critical role in the accurate accounting of derelict spacecraft and space debris, and in the tracking of active, operational spacecraft that could pose a risk to national interests. As countries continue to develop spacecraft designed for close-proximity and on-orbit servicing operations, advancing space situational awareness provides an important way to mitigate any potential threats.
References
1. Joint Chiefs of Staff. Space Operations (Joint Publication No. 3-14). (2018, April). U.S. Department of Defense. Retrieved from http://www.jcs.mil/Portals/36/Documents/Doctrine/pubs/jp3_14.pdf
2. Space-Track. (n. d.). SAIC. Retrieved from https://www.space-track.org/
3. European Space Agency. (2018, February). Space debris. Retrieved from https://www.esa.int/Our_Activities/Operations/Space_Debris/About_space_debris
4. Liou, J-C. (2018, February). USA Space debris environment, operations, and research updates. Paper presented at the 55th Session of the Scientific and Technical Subcommittee Committee on the Peaceful Uses of Outer Space, United Nations, Vienna, AUT. Retrieved from https://ntrs.nasa.gov/archive/nasa/casi.ntrs.nasa.gov/20180001749.pdf
5. European Space Agency. (2018, January). Space debris by the numbers. Retrieved from https://www.esa.int/Our_Activities/Operations/Space_Debris/Space_debris_by_the_numbers
6. NASA. (2018, April). Handbook for Limiting Orbital Debris (NASA-HANDBOOK 8719.14). Washington, D.C. Retrieved from https://standards.nasa.gov/standard/nasa/nasa-hdbk-871914
7. Regeon, P. A. (2002, September 30). Military Global Positioning System (GPS) Augmentation System (MGAS). Arlington, VA: Office of Naval Research. Retrieved from http://www.dtic.mil/dtic/tr/fulltext/u2/a630533.pdf
8. Federal Aviation Administration. (2018, June 26). Satellite Navigation – Wide Area Augmentation System (WAAS). Retrieved from https://www.faa.gov/about/office_org/headquarters_offices/ato/service_units/techops/navservices/gnss/waas/
9. Air Force Space Command. (2016, December).Space based infrared system. Retrieved from http://www.afspc.af.mil/About-Us/Fact-Sheets/Display/Article/1012596/space-based-infrared-system/
10. Air Force Space Command. (2017, March 22). Geosynchronous space situational awareness program. Retrieved from http://www.afspc.af.mil/About-Us/Fact-Sheets/Article/730802/geosynchronous-space-situational-awareness-program-gssap/
11. Brew, J., & Holzinger, M. (2018). Probabilistic resident space object detection using archival THEMIS fluxgate magnetometer data. Advances in Space Research, 61(9),2301–2319. doi:10.1016/j.asr.2018.01.045
12. Becker, D., & Cain, S. (2018). Improved space object detection using short-exposure image data with daylight background. Applied Optics, 57(14), 3968–3975.doi:10.1364/AO.57.003968
13. Vallduriola, G., Scharf, A., Pittet, J., Utzmann, J., Trujillo, D., Vananti, A., . . . Daens, D. (2018, June). The use of different architectures and streak observations algorithms to detect space debris. ARCS Workshop 2018; 31th International Conference on Architecture of Computing Systems. Retrieved from https://ieeexplore.ieee.org/document/8385435/
14. Freedman, J., & Halvorson, E. (2018). U.S. Patent No. 9916507. Washington, DC: U.S. Patent and Trademark Office.
15. Chow, B. G. (2018, Summer). Space arms control: A hybrid approach. Strategic Studies Quarterly. Retrieved from http://www.airuniversity.af.mil/Portals/10/SSQ/documents/
Volume-12_Issue-2/Chow.pdf
16. Air Force Office of Scientific Research. (2018/12/15-2020/12/14). URED Project Abstract: C1160 An Integrated Approach to Space Situational Awareness. EF128595.
17. Air Force Office of Scientific Research. (2015/08/15-2019/02/14). URED Project Abstract: Innovations in Statistical Image Analysis and Applications to 3D Imaging for Improved SSA. EF126284.
18. Air Force Office of Scientific Research. (2016/04/15-2019/04/14). URED Project Abstract: Advanced Orbit Prediction for Resident Space Objects through Physics-based Learning. EF127426.
19. Air Force Office of Scientific Research. (2017/12/05-2018/12/14). URED Project Abstract: STARBROOK EXPRESS Dynamic Calibration of Sensor Measurements for Near Real-time Space Object Tracking and Characterization. EF130266.
20. Air Force Office of Scientific Research. (2015/12/01-2017/11/30). URED Project Abstract: Finite Set Statistics on Manifolds for Space Object. EF127106.
21. Air Force Office of Scientific Research. (2015/07/15-2018/07/14). URED Project Abstract: Optimal Sensor Tasking for Space Situational Awareness. EF126320.
22. Small Business Innovation Research (SBIR) Program. (2017). Rapid Discovery of Evasive Satellite Behaviors. Topic Number AF17-CT02. Retrieved from https://www.sbir.gov/sbirsearch/detail/1319195
23. Nastasi, K. M., & Black, J. (2018). An autonomous sensor management strategy for monitoring a dynamic space domain with diverse sensors. 2018 AIAA Information Systems-AIAA Infotech @ Aerospace (AIAA 2018-0890). doi:10.2514/6.2018-0890
24. Faber, W. R., Hussein, I. I., Kent, J. T., Bhattacharjee, S., & Jah, M. (2018). Optical data processing using directional statistics in a multiple hypothesis framework with maneuvering objects. 2018 AIAA Information Systems-AIAA Infotech @ Aerospace (AIAA 2018-1971). doi:10.2514/6.2018-1971
25. Liou, J.-C. (2018). USA space debris environment, operations, and research updates. Presented at the 55th Session of the Scientific and Technical Subcommittee Committee on the Peaceful Uses of Outer Space, United Nations, Vienna. Retrieved from https://ntrs.nasa.gov/archive/nasa/casi.ntrs. nasa.gov/20180001749.pdf
26. NASA. (n.d.). Astromaterials Research & Exploration Science. Retrieved from https://orbitaldebris.jsc.nasa.gov/photo-gallery.html